b Jiangsu Optoelectronic Functional Materials & Engineering Laboratory, Nanjing 211189, China
The counter electrode (CE) plays a very important role in dyesensitized solar cells (DSSC). In general,DSSC are comprised of a dye-sensitized TiO2 mesoporous film-coated,fluorine-doped tin oxide transparent glass (FTO) substrate as a working electrode, triiodide/iodide (I3-/I-) redox couple in organic solutions as a liquid electrolyte,and a platinized FTO as a counter electrode. The role of Pt-CE is to collect Rss from the external circuit and reduce I3- to I- at the surface of CE. The electrocatalytic reduction from I3- to Iaffects the dye regeneration rate at the working electrode,which suggests the photocurrent of DSSC can be influenced by the electrochemical activity of the CE. So far,Pt-CE has been widely used for DSSC owing to their superior catalytic activity in the reduction of I3-. As a precious metal with limited supply,Pt has been replaced by other materials,including carbon-based materials [1, 2, 3],conducting polymers [4, 5],and early transition metals (carbides,nitrides and oxides) [6, 7]. In particular,the modified multi-wall carbon nanotube (MWCNT) is often considered as a potential alternative CE for DSSC [8, 9, 10],due to its low cost,high catalytic activity in I3- reduction,and excellent corrosion resistance toward the liquid electrolytes. Compared with Pt,the inferior electrocatalytic activity of MWCNT in I3- reduction limits its application as a CE for the high performance DSSC. In order to understand the factors that influence the I3- reduction process, many electrochemical methods can be used to delineate the reaction process from I3- to I-,such as cyclic voltammetry (CV), electrochemical impedance spectroscopy (EIS) and Tafel curve.
Herein,we report a facile,low-cost and scalable preparation of CE by mixing different proportions of MWCNT and TiO2. The performance of DSSC based on MWCNT-TiO2 CE was investigated to optimize the composition of hybrid materials. The redox ability of various CEs was determined by CV,such as the anodic and cathodic peak potential (Ep,a and Ep,c),and the anodic and cathodic peak current density (ip,a and ip,c). Furthermore,the electrochemical catalytic activity was characterized by EIS and Tafel measurements, including the exchange current density (J0),the limiting diffusion current density (Jlim),and the diffusion coefficient of I3- and I- (Dtri and Di) in liquid electrolytes. 2. Experimental 2.1. Preparation of MWCNT-TiO2 counter electrode
The mixture of MWCNT (S1020,Shenzhen Nanotech Port), ethylcellulose and TiO2 (P25,Desgussa) (mass ratio10:2:x,x = 2,4,5,6,7) was dispersed into terpineol by repeated grinding,stirring and ultrasound radiation. The paste was coated onto FTO (sheet resistance 10 Ω/square,Asahi) substrate through the doctorblading technique. After it was sintered at 350℃ for 1 h,the MWCNT-TiO2 counter electrode was produced and labeled as S1, S2,S3,S4 and S5 with various components of MWCNT and TiO2. 2.2. Assembly of DSSC
The working electrode was prepared and sensitized as reported previously [11, 12]. The composition of electrolyte was as follows: 0.5 mol/L LiI,0.05 mol/L I2 and 0.05 mol/L 4-tert-butylpyridine in propylene carbonate. The N3-sensitized (N3,Solaronix) working electrode was assembled with the MWCNT-TiO2 counter electrode using hot-melt sealing glue (Surlyn,DuPont). The electrolyte was injected into the gap of DSSC through a micro-hole in the counter electrode with the help of a vacuum back-filling system. The hole was subsequently sealed with a microscopic coverslide and a sealing sheet. The working electrode with a shadow mask of 0.25 cm2 was defined as an active area of the cell. 2.3. Measurements
The morphology of CE was observed using a field-emission scanning Rs microscope (FE-SEM,FEI). Photocurrent density-voltage curves were measured by a Keithley2400 under Oriel solar simulator (AM 1.5,100 mW/cm2) illumination. All of the electrochemical characterizations were carried out using a CHI760D electrochemical workstation. CV was measured in a three-electrode electrochemical cell using MWCNT-TiO2 as a working electrode,Pt wire as a counter electrode and saturated calomel electrode (SCE) as a reference electrode in a 0.1 mol/L LiClO4,0.01mol/L LiI and 0.001mol/L I2 acetonitrile solution. The scan rate was 50 mV/s. EIS and Tafel measurements were carried out in a symmetrical cell by sandwiching two identical counter electrodes face-to-face and filling with the electrolyte in a similar manner to DSSC assembly. EIS was recorded in a frequency range of 1 × 10-1 - 1 × 105 Hz with the open-circuit voltage (Voc) of DSSC as the applied bias voltage and 10 mV as ac amplitude in dark. The impedance data were fitted with ZSimpWin software. Tafel measurements were carried out at a scan rate of 5 mV/s. 3. Results and discussion
Fig. 1 shows the morphology of MWCNT-TiO2 hybrid materials onto the FTO substrates with different ratio. With the increase of the amount of TiO2,the adhesion ability between MWCNT-TiO2 and FTO was enhanced so that the hybrid film might not be easy to detach,suggesting the efficient Rs transfer from the catalytic sites of hybrid materials to the FTO substrates. Meanwhile,the rough and porous surface is conducive to the development of electrolyte transfer,which can provide a large number of diffusion channels for liquid electrolytes. However,the TiO2 addition also can cause the decrease the number of catalytic sites on the MWCNT-TiO2 surfaces since TiO2 occupies some active centers on the MWCNT surface. Therefore,the appropriate TiO2 ratio needs to be considered carefully.
![]() |
Download:
|
Fig. 1.Morphologies of MWCNT-TiO2 distributed on FTO substrates: (a-f) indicating MWCNT and S1-S5. |
The redox properties of hybrid materials were investigated by CV plots. As for Pt,two pairs of redox peaks are attributed to four sequential reactions,as shown in Fig. 2a. The first cathodic peak at low potential and the second cathodic peak at high potential represent the consumption and generation of I3-,respectively. Compared with Pt,the similar cathodic peak potential and the approximate peak current density were also found in the CV plots of the pure MWCNT as a CE. This indicates that the MWCNT-based materials are a potential substitute material for Pt as a counter electrode. Fig. 2b shows the CV plots of various MWCNT-TiO2 electrodes,where the redox potentials and the peak current responses change slightly. All CV parameters are listed in Table 1. Furthermore,Dtri and Di can be estimated by the Randles-Sevcik equation [13]:

![]() |
Download:
|
Fig. 2.CV plots of I3- species in (a) Pt,MWCNT and (b) S1-S5 electrodes. |
![]() |
Table 1 Results derived from the CV plots. |
Fig. 3a shows the photocurrent density versus voltage curves for DSSC using Pt,MWCNT,and S1-S5 as counter electrodes. In theory,Voc is determined by the difference between the potential of I3-/I- species and the quasi-Fermi level of Rss in TiO2 working electrode. The range of Voc is from 0.69 Ω to 0.75 V,where the distribution is in the range of 0.72 Ω plus or minus 0.03 V. In addition to the measurement error,the main reason for this difference is that the potential of I3-/I- species versus various CEs has a certain level of variability. Although the hybrid materials were prepared by simple mixing and sintering,the tiny change might influence the Rsic structure of the material surface. Therefore,this effect will result in a shift of Voc. In general,the short-circuit photocurrent density (Jsc) denotes the internal effective Rs transferring ability in DSSC. The Jsc of S3-DSSC is second only to that of Pt-DSSC as a counter electrode. This phenomenon is in accordance with the diffusion effect of redox couples,owing to the fact that the high diffusion coefficient might help the dye-regeneration. The fill factor (FF) expresses the electric power capacity on the outside of DSSC. The FF of MWCNT-DSSC is lower than that of the other DSSC. In the process of assembling MWCNT-DSSC,the MWCNT film was easy to detach due to the slack adhesion with FTO substrates. This results in the increases of the Rs transport barrier from MWCNT to FTO. The comprehensive performance of DSSC is the photon-to-Rs conversion efficiency (η),which can be calculated by Voc,Jsc,FF and intensity of incident light (Pin) using Eq. (2):

![]() |
Download:
|
Fig. 3.(a) J-V curves and (b) Nyquist plots. Insert: equivalent circuit diagram. |
![]() |
Table 2 Photovoltaic parameters of DSSC and electrochemical data of symmetric cell. |
To further evaluate the electrochemical catalytic activity of different CEs,Tafel curves were also carried out in a dummy cell similar to the one used in EIS,as shown in Fig. 4a. The anodic and cathodic branches of the logarithmic current density (Log J) versus voltage can be divided into polarization zone,Tafel zone and limit diffusion zone from the low potential to high potential in turn. The latter two zones can provide J0 and Jlim,which measure the catalytic activity of CE. In the Tafel zone,J0 can be obtained as the intersection of the extrapolated linear anodic and cathodic branches,which is a kinetic parameter based on the redox reaction and the electrode surface state. Therefore,J0 also can be calculated from Rct according to EIS results using Eq. (3). In the limit diffusion zone,Jlim is determined by the diffusion of the redox species between the two identical electrodes,which might have a close relationship with the diffusion impedance from EIS and diffusion coefficient from CV. Briefly,Jlim is proportional to Dtri as described by Eq. (4).
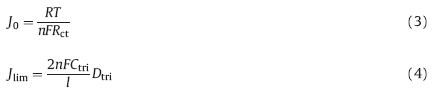
![]() |
Table 3 Results from different electrochemical methods. |
![]() |
Download:
|
Fig. 4.Tafel curves of (a) the three-zone diagram and (b) various counter electrodes. |
In conclusion,we have developed and investigated a simple and feasible method for preparing a potential alternative MWCNT- TiO2 CE for DSSC. Compared with the Pt-CE,DSSC based on the MWCNT-TiO2 (mass ratio of 2:1) CE can obtain the optimum photovoltaic performance due to its low Rs and Rct,and high Dtri. Moreover,the reduction process from I3- to I- is determined by the kinetic-controlled and diffusion-limited processes. Webelieve that the reasonable regulation can be very beneficial to the design and preparation of a practical CE for DSSC according to the reduction of I3-. Acknowledgments
We are grateful for the grants from the National Natural Science Foundation of China (No. 21173042),National Basic Research Program of China (No. 2013CB932902),Fundamental Research Funds for the Central Universities (No. 3207043401),Science & Technology Support Project of Jiangsu (No. BE2013118),and Jiangsu Key Laboratory of Environmental Material & Environmental Engineering (No. JHCG201012).
[1] | B. Lee, D.B. Buchholz, R. Chang, An all carbon counter electrode for dye-sensitized solar cells, Energy Environ. Sci. 5 (2012) 6941-6952. |
[2] | G.T. Yue, J.H. Wu, J. Lin, et al., A counter electrode of multi-wall carbon nanotubes decorated with tungsten sulfide used in dye-sensitized solar cells, Carbon 55 (2013) 1-9. |
[3] | S. Das, P. Sudhagar, V. Verma, et al., Amplifying charge-transfer characteristics of graphene for triiodide reduction in dye-sensitized solar cells, Adv. Funct. Mater. 21 (2011) 3729-3736. |
[4] | P. Sudhagar, S. Nagarajan, Y. Lee, et al., Synergistic catalytic effect of a composite (CoS/PEDOT:PSS) counter electrode on triiodide reduction in dye-sensitized solar cells, ACS Appl. Mater. Interfaces 3 (2011) 1838-1843. |
[5] | J. Zhang, T. Hreid, X.Y. Li, et al., Nanostructured polyaniline counter electrode for dye-sensitised solar cells: fabrication and investigation of its electrochemical formation mechanism, Electrochim. Acta 55 (2010) 3664-3668. |
[6] | M.X. Wu, X. Lin, Y.D. Wang, et al., Economical Pt-free catalysts for counter electrodes of dye-sensitized solar cells, J. Am. Chem. Soc. 134 (2012) 3419-3428. |
[7] | Y.D. Wang, M.X. Wu, X. Lin, et al., Several highly efficient catalysts for Pt-free and FTO-free counter electrodes of dye-sensitized solar cells, J. Mater. Chem. 22 (2012) 4009-4014. |
[8] | Y. Xiao, J. Lin, S.Y. Tai, et al., Pulse electropolymerization of high performance PEDOT/MWCNT counter electrodes for Pt-free dye-sensitized solar cells, J. Mater. Chem. 22 (2012) 19919-19925. |
[9] | P. Joshi, Y. Xie, M. Ropp, et al., Dye-sensitized solar cells based on low cost nanoscale carbon/TiO2 composite counter electrode, Energy Environ. Sci. 2 (2009) 426-429. |
[10] | K. Huang, Y. Wang, R. Dong, et al., A high performance dye-sensitized solar cell with a novel nanocomposite film of PtNP/MWCNT on the counter electrode, J. Mater. Chem. 20 (2010) 4067-4073. |
[11] | Y.Q. Wang, Y.M. Sun, B. Song, J.T. Xi, Ionic liquid electrolytes based on 1-vinyl-3- alkylimidazolium iodides for dye-sensitized solar cells, Sol. Energy Mater. Sol. Cells 92 (2008) 660-666. |
[12] | Y.R. Gao, L.L. Chu, W. Guo, L.T. Ma, Synthesis and photoelectric properties of an organic dye containing benzo[1,2-b:4,5-b 0]dithiophene for dye-sensitized solar cells, Chin. Chem. Lett. 24 (2013) 149-152. |
[13] | Y.T. Tang, X. Pan, C.N. Zhang, et al., Influence of different electrolytes on the reaction mechanism of a triiodide/iodide redox couple on the platinized FTO glass electrode in dye-sensitized solar cells, J. Phys. Chem. C 114 (2010) 4160-4167. |