2. 上海海洋大学 农业农村部淡水水产种质资源重点实验室, 上海 201306;
3. 上海海洋大学 水产科学国家级实验教学示范中心, 上海 201306
鱼类在野外生存和养殖过程中常会受到饥饿或营养不良的影响[1]。因此,饥饿是鱼类生活史中经常面临的环境胁迫因子之一,受饥饿影响的鱼类会在其行为、形态和生理等多方面做出一些适应性的调节[2], 如饥饿导致鱼类渗透调节能力降低[3],鳃小片缩短或肥大[4],减少鳃氯细胞的大小和数量[5]。鳃是鱼类主要的呼吸器官,具有气体交换、离子平衡和渗透压调节等重要功能[6],PERRY等[7]发现,罗非鱼(Oreochromis spp)鳃氯细胞密度与Na+/K+-ATP酶活性直接相关。尽管目前对鱼鳃缺乏了解[8-9],但这个器官的健康对鱼的生存无疑是至关重要的。
团头鲂(Megalobrama amblycephala)隶属于鲤形目(Cypriniformes)鲤科(Cyprinidae)鲂属(Megalobrama),俗称武昌鱼,系草食性经济鱼类,具有肉质鲜美、含肉率高、食性广、生长快和抗病力强等特点,是中国主要的淡水养殖对象之一[10],2018年的全国产量约83万t[11]。目前,饥饿胁迫对团头鲂的影响研究主要集中在生长、生理生化、代谢、免疫和疾病上[12-14]。饥饿和再投喂对其鳃组织结构和酶活性影响的研究还未见报道。本研究利用光镜和扫描电镜观察了饥饿和恢复投喂状态下团头鲂耐低氧F4幼鱼鳃的组织学和超微结构,同时,测定了其鳃组织中超氧化物歧化酶(superoxide dismutase, SOD)、过氧化氢酶(catalase from micrococcus lysodeikticus, CAT)和钠钾ATP酶(sodium potassium ATPase, Na+/K+-ATP)的活性,对于认识团头鲂生理学和指导团头鲂养殖生产具有重要意义。
1 材料与方法 1.1 实验材料实验材料为上海海洋大学农业农村部团头鲂遗传育种中心繁殖的团头鲂耐低氧F4,体质量(30.5±2.6)g。将团头鲂耐低氧F4暂养在室内规格为35 cm×55 cm×45 cm的塑料水族箱中,温度(temperature,T)为(25±1.0)℃,溶解氧(dissolved oxygen,DO)为(7.0±0.5)mg/L,pH为7.5±0.3,使用经曝气的自来水,每日吸污换水1/3,每日投喂2次(8:00,17:00),保持自然光照。
1.2 实验设计将30尾团头鲂耐低氧F4幼鱼放在恒温液浴循环槽[DO=(7.0±0.5)mg/L,T=(25±1.0)℃]中暂养1周后开始禁食,实验设3组平行,分别在饥饿0、5、10、15、20、25和30 d以及恢复投喂7 d时,从各平行组中取3尾鱼,取鳃组织做切片,扫描电镜观察和酶活性测定。
1.3 光学显微镜和扫描电子显微镜观察在饥饿0、5、10、15、20、25和30 d以及恢复投喂7 d时,随机取3尾团头鲂耐低氧F4幼鱼,用0.5 g/L的MS-222麻醉后活体冰上解剖,从右侧第二鳃弓上取下鳃片作为光学显微镜观察样本,第三鳃弓上取下鳃片作为扫描电子显微镜观察样本,在0.75%的生理盐水中反复润洗,以清除取样时黏附在鳃丝上的血细胞和黏液。进行光学显微镜观察的鳃组织放入Boiun氏液中固定24 h,常规脱水,二甲苯透明,石蜡包埋,连续切片(厚度5 μm),经H.E染色后在显微镜下观察并拍照。进行扫描电子显微镜观察的鳃组织放入2.5%戊二醛中固定24 h(4 ℃),用磷酸缓冲溶液漂洗3次,再用1%锇酸固定1 h(4 ℃),用磷酸缓冲溶液漂洗,50%~100%乙醇逐级脱水,经醋酸异戊酯置换后常规临界干燥,真空离子镀膜,在Hitachi S-3400N型扫描电镜下观察、拍照。
1.4 Na+/K+-ATP和抗氧化酶测定取饥饿0、5、10、15、20、25和30 d以及恢复投喂7 d时的团头鲂耐低氧F4幼鱼鳃组织,按质量(g):体积(mL)=1: 9的比例加入9倍体积的0.86%的生理盐水于离心管中,制备匀浆液,2 500 r/min,离心10 min,取上清液,采用南京建成生物公司的检测试剂盒测定鳃组织中CAT、SOD及Na+/K+-ATP酶的活性。
1.5 鳃组织形态学测量为了评估团头鲂耐低氧F4鳃小片呼吸面积和层间基质(interlamellar cell mass,ILCM)体积的变化,进行以下形态测量:(1)伸出鳃小片基部的长度;(2)鳃小片厚度;(3)伸出鳃小片的长度。每个参数在同一饥饿时间进行30次测量。鳃小片面积以近似为半椭圆计算[15],公式为

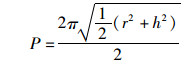


式中:a是鳃小片呼吸面积, μm2; l是鳃小片基部的长度, μm; p是椭圆的周长, μm; h是鳃小片伸出的长度, μm; t是鳃小片厚度, μm; V是鳃小片ILCM体积, μm3; d是相邻两鳃小片间距离, μm; Hi是鳃小片ILCM厚度, μm。
1.6 数据分析所得数据均用平均值±标准差(Mean±SD)表示,不同处理组数据间的差异性采用方差分析,P < 0.05为存在显著性差异。
2 结果 2.1 饥饿胁迫对团头鲂耐低氧F4鳃组织结构的影响在T=(25±1.0)℃,DO=(7.0±0.5)mg/L条件下,对团头鲂耐低氧F4进行饥饿和恢复投喂研究。分别取团头鲂耐低氧F4饥饿0、5、10、15、20、25和30 d以及恢复投喂7 d时的鳃组织,通过光镜和扫描电镜观察发现:饥饿0 d时,团头鲂耐低氧F4伸出鳃小片的长度为(58.5±0.3)μm,饥饿30 d时为(82.6±0.6)μm,增加了41.2%,差异显著(P < 0.05,表 1, 图版Ⅰ-Ⅱ)。由于伸出鳃小片长度增加,进而导致鳃小片平均呼吸面积增加。饥饿0 d时,团头鲂耐低氧F4鳃小片呼吸面积(12 549 μm)与饥饿30 d时(36 211 μm)相比,增加了188.6%,差异显著(P < 0.05,图 1a)。同时,鳃小片层间基质的厚度减少,导致层间基质的体积减少。饥饿0 d时,团头鲂耐低氧F4相邻两鳃小片层间基质的平均厚度(28.6±1.2) μm与饥饿30 d(18.6±0.2) μm相比,减少了53.8%,差异显著(P < 0.05,表 1, 图版Ⅰ-Ⅱ)。饥饿0 d团头鲂耐低氧F4鳃小片ILCM体积为(24 634 μm),饥饿30 d时为(10 282 μm),减少了139.6%,差异显著(P < 0.05,图 1b)。恢复投喂7 d后,团头鲂耐低氧F4鳃组织基本恢复到正常投喂时的形态(表 1,图版Ⅰ-Ⅱ)。
![]() |
表 1 在饥饿胁迫下团头鲂耐低氧F4鳃组织的形态学特征 Tab.1 Morphometric characteristics of hypoxia-tolerant F4 generation of blunt snout bream gills under hunger stress |
![]() |
图中1-8分别表示团头鲂耐低氧F4鳃组织在饥饿胁迫0、5、10、15、20、25和30d以及恢复投喂7 d时的形态变化。其中,黑色箭头表示鳃小片,红色箭头表示相邻两鳃小片ILCM,H.E染色 Picture series starts with 0 day of hunger (1), 5, 10, 15, 20, 25, 30 days of hunger (2-7)and 7 days of recovery (8).Black arrows is lamellae, Red arrows reveal ILCM. H.E. Staining 图版 Ⅰ 饥饿和恢复投喂状态下团头鲂耐低氧F4鳃组织的显微结构 Plate Ⅰ Light microscope (LM) micrographs of the gill lamellae of hypoxia-tolerant F4generation of blunt snout bream during hunger and re-feeding |
![]() |
图版中1-8分别表示团头鲂耐低氧F4鳃组织在饥饿胁迫0、5、10、15、20、25和30 d以及恢复投喂7 d时的形态变化 Picture series starts with 0 day of hunger (1), 5, 10, 15, 20, 25, 30 days of hunger (2-7), and 7 days of recovery (8) 图版 Ⅱ 饥饿和恢复投喂状态下团头鲂耐低氧F4鳃组织的超微结构 Plate Ⅱ Scanning electron microscopy (SEM) micrographs of the gill lamellae of hypoxia-tolerant F4generation of blunt shout bream during hunger and re-feeding |
![]() |
图中1-8分别表示团头鲂耐低氧F4饥饿胁迫0、5、10、15、20、25和30 d以及恢复投喂7 d。不同的小写字母表示显著差异(P < 0.05) Picture series starts with 0 days of hunger (1), 5, 10, 15, 20, 25, 30 days of hunger (2-7), 7 days of recovery(8).Letters that differ indicate statistically significant differences (P < 0.05) 图 1 在饥饿胁迫下团头鲂耐低氧F4鳃小片平均面积(a)和相邻两鳃小片ILCM体积(b) Fig. 1 Mass-specific lamellar area (a) and ILCM volume (b) change of hypoxia-tolerant F4 generation of blunt snout bream under hunger treatment |
饥饿和恢复投喂对团头鲂耐低氧F4幼鱼鳃组织的Na+/K+-ATP酶和抗氧化酶影响见表 2。从表 2可以看出,随着饥饿时间的延长,团头鲂耐低氧F4鳃组织中的Na+/K+-ATP、SOD和CAT酶活性降低。在饥饿0 d时,团头鲂耐低氧F4鳃的Na+/K+-ATP、SOD和CAT酶活性分别为(2.21±0.08)U/mg、(17.31±0.04)U/mg和(5.77±0.02)U/mg,在饥饿30 d时分别为(1.78±0.01)U/mg、(15.82±0.04)U/mg和(4.66±0.16)U/mg,分别降低了24.2%,9.4%和23.8%,差异显著(P < 0.05)。恢复投喂7 d,基本恢复到正常水平(表 2)。
![]() |
表 2 在饥饿胁迫下团头鲂耐低氧F4幼鱼鳃组织的氧化应激反应 Tab.2 Effects of hunger stress on immunoenzyme enzyme activity of the gills of hypoxia-tolerant F4 generation of blunt shout stress |
鳃是鱼类呼吸、氨氮排泄及渗透调节的主要部位。研究表明,食物中缺乏维生素会导致奥利亚罗非鱼(Oreochromis aureus)[16],虹鳟(Oncorhynchus mykiss)[17],大麻哈鱼(Oncorhynchus rhodurus)[18],胡子鲇(Clarias gariepinus)[19]等鳃小片上皮细胞增生、水肿和毛细血管扩张。团头鲂在缺氧水环境中表现出鳃的重构现象,保留了应对缺氧和温度升高的能力[20]。通过鳃组织切片观察发现, 团头鲂耐低氧F4在饥饿条件下,鳃小片平均伸出长度增加,层间基质的厚度减小,导致鳃小片呼吸面积增加和ILCM体积减小,说明鳃主动调节机制被激活。
生物体内存在一套完整的抗氧化防御系统,其中,SOD是已知生物体内唯一以自由基为底物的酶,催化超氧阴离子发生歧化反应转变为H2O2和O2[21],随后由CAT和其他酶协同作用,催化H2O2分解为H2O和O2[22-23]。当水体中胁迫强度低于鱼体耐受限度时, 机体能自行调节从而适应外界环境变化[24]。当受到连续刺激且超过机体调节阈值时, 机体抗氧化系统受到破坏, 部分抗氧化物质含量及酶活性下降, 机体清除自由基的能力降低[25], 脂质过氧化产物增多, 从而破坏机体非特异性免疫防御系统, 导致免疫力下降[26-27]。研究发现在一些鱼类中,如褐鳟(Salmo trutta)[28]、滨岸护胸鲇(Hoplosternum littorale)[29]和大西洋鲷(Sparus aurata)[30],饥饿会引起其体内抗氧化相关酶活性的变化,这表明机体受到了饥饿造成的氧化压力。团头鲂耐低氧F4鳃组织中SOD酶活力和CAT酶活力与饥饿0 d相比,显著降低(P < 0.05)。这可能是由于饥饿胁迫破坏了团头鲂耐低氧F4的抗氧化系统,SOD和CAT酶活性下降,降低了自身清除自由基的能力,因此饥饿胁迫使团头鲂耐低氧F4鳃中脂类氧化所产生的过多ROS反过来抑制了酶活性。恢复投喂7 d后,团头鲂耐低氧F4鳃组织中SOD和CAT酶活性基本上恢复正常水平,表明在长时间的饥饿条件下,团头鲂耐低氧F4能够通过自身的调节维持基本生命活力。
Na+/K+-ATP酶是鳃组织泌氯细胞及细胞器的膜上存在的一种蛋白酶, 在鱼体渗透调节过程中起着非常重要的作用[31]。莫桑比克罗非鱼(Oreochromis mossambicus)[5]和虹鳟[32]鳃组织的Na+/K+-ATP酶活性在饥饿条件下均显著降低。饥饿胁迫30 d,团头鲂耐低氧F4鳃丝Na+/K+-ATP酶活性比饥饿0 d显著降低(P < 0.05), 当恢复投喂7 d后,其酶活性基本恢复到正常水平。其原因可能是, 在饥饿胁迫下,没有足够的营养物质,机体内缺乏氨基酸使得Na+/K+-ATP酶蛋白结构受到影响而致酶活力降低, 然后机体渗透机制被有效激活进行主动渗透调节[33]。
[1] |
PÉREZ-JIMÉNEZ A, GUEDES M J, MORALES A E, et al. Metabolic responses to short starvation and refeeding in Dicentrarchus labrax. Effect of dietary composition[J]. Aquaculture, 2007, 265(1/4): 325-335. |
[2] |
MCCUE M D. Starvation physiology:reviewing the different strategies animals use to survive a common challenge[J]. Comparative Biochemistry and Physiology Part A:Molecular & Integrative Physiology, 2010, 156(1): 1-18. |
[3] |
STEFANSSON S O, IMSLAND A K, HANDELAND S O. Food-deprivation, compensatory growth and hydro-mineral balance in Atlantic salmon (Salmo salar) post-smolts in sea water[J]. Aquaculture, 2009, 290(3/4): 243-249. |
[4] |
WOOD E M, YASUTAKE W T. Histopathology of fish:Ⅴ. Gill disease[J]. The Progressive Fish-Culturist, 1957, 19(1): 7-13. DOI:10.1577/1548-8659(1957)19[7:HOF]2.0.CO;2 |
[5] |
KÜLTZ D, JÜRSS K. Acclimation of chloride cells and Na/K-ATPase to energy deficiency in tilapia (Oreochromis mossambicus)[J]. Zoologische Jahrbucher Abteilung fur Allgemeine Zoologie und Physiologie der Tiere, 1991, 95: 39-50. |
[6] |
刘凌云, 郑光美. 普通动物学[M]. 4版. 北京: 高等教育出版社, 2009: 343. LIU L Y, ZHENG G M. General zoology[M]. 4th ed. Beijing: Higher Education Press, 2009: 343. |
[7] |
PERRY S F, WALSH P J. Metabolism of isolated fish gill cells:contribution of epithelial chloride cells[J]. Journal of Experimental Biology, 1989, 144: 507-520. |
[8] |
OLSON K R. Gill circulation:regulation of perfusion distri-bution and metabolism of regulatory molecules[J]. Journal of Experimental Zoology, 2002, 293(3): 320-335. DOI:10.1002/jez.10126 |
[9] |
FERGUSON W H. Systemic pathology of fish:a text and atlas of normal tissues in teleosts and their responses in disease[M]. 2nd ed. London: Scotian Press, 2006.
|
[10] |
LI S F, CAI W Q, ZHOU B Y. Variation in morphology and biochemical genetic markers among populations of blunt snout bream (Megalobrama amblycephala)[J]. Aquaculture, 1993, 111(1/4): 117-127. |
[11] |
农业农村部渔业渔政管理局. 中国渔业统计年鉴[M]. 北京: 中国农业出版社, 2018. Fisheries and Fisheries Administration Bureau of the Ministry of Agriculture and Rural Areas. China fishery statistical year book[M]. Beijing: China Agriculture Press, 2018. |
[12] |
苏艳莉, 戈贤平, 孙盛明, 等. 高温季节饥饿后补偿摄食对团头鲂幼鱼生长性能、血清生化指标、肠道消化酶活性及肝脏抗氧化酶活性的影响[J]. 动物营养学报, 2017, 29(11): 4198-4206. SU Y L, GE X P, SUN S M, et al. Effects of compensatory feeding after starvation on growth performance, serum biochemical indexes, intestinal digestive enzyme activities and hepatic antioxidant enzyme activities of juvenile blunt snout bream (Megalobrama amblycephal) in summer[J]. Chinese Journal of Animal Nutrition, 2017, 29(11): 4198-4206. DOI:10.3969/j.issn.1006-267x.2017.11.044 |
[13] |
REN M C, HABTE-TSION H M, LIU B, et al. Food depri-vation of blunt snout bream, Megalobrama amblycephala fingerlings and the subsequent effect of feeding with different dietary starch levels on glucose metabolism[J]. The Israeli journal of aquaculture-Bamidgeh, 2015, 67: 1-9. |
[14] |
LI X F, TIAN H Y, ZHANG D D, et al. Feeding frequency affects stress, innate immunity and disease resistance of juvenile blunt snout bream Megalobrama amblycephala[J]. Fish & Shellfish Immunology, 2014, 38(1): 80-87. |
[15] |
SOLLID J, DE ANGELIS P, GUNDERSEN K, et al. Hy-poxia induces adaptive and reversible gross morphological changes in crucian carp gills[J]. Journal of Experimental Biology, 2003, 206(20): 3667-3673. DOI:10.1242/jeb.00594 |
[16] |
SOLIMAN A K, WILSON R P. Water-soluble vitamin requi-rements of tilapia. 1 Pantothenic acid requirement of blue tilapia, Oreochromis aureus[J]. Aquaculture, 1992, 104(1/2): 121-126. |
[17] |
OLSVIK P A, HEMRE G I, WAAGBØ R. Exploring early micronutrient deficiencies in rainbow trout (Oncorhynchus mykiss) by next-generation sequencing technology-from black box to functional genomics[J]. PLoS One, 2013, 8(7): e69461. DOI:10.1371/journal.pone.0069461 |
[18] |
TAVEEKIJAKARN P, MIYAZAKI T, MATSUMOTO M, et al. Studies on vitamin K deficiency in amago salmon, Oncorhynchus rhodurus(Jordan & McGregor)[J]. Journal of Fish Diseases, 1996, 19(3): 209-214. DOI:10.1111/j.1365-2761.1996.tb00127.x |
[19] |
ADHAM K G, HASHEM H O, ABU-SHABANA M B, et al. Vitamin C deficiency in the catfish Clarias gariepinus[J]. Aquaculture Nutrition, 2000, 6(2): 129-139. DOI:10.1046/j.1365-2095.2000.00139.x |
[20] |
WU C B, LIU Z Y, LI F G, et al. Gill remodeling in response to hypoxia and temperature occurs in the hypoxia sensitive blunt snout bream (Megalobrama amblycephala)[J]. Aquaculture, 2017, 479: 479-486. DOI:10.1016/j.aquaculture.2017.06.020 |
[21] |
KŁUCIŃSKI P, WÓJCIK A, GRABOWSKA-BOCHENEK R, et al. Erythrocyte antioxidant parameters in workers occupationally exposed to low levels of ionizing radiation[J]. Annals of Agricultural and Environmental Medicine, 2008, 15(1): 9-12. |
[22] |
吴志昊, 尤锋, 王英芳, 等. 低氧和高氧对大菱鲆幼鱼红细胞核异常及氧化抗氧化平衡的影响[J]. 上海海洋大学学报, 2011, 20(6): 808-813. WU Z H, YOU F, WNAG Y F, et al. The effects of hypoxia and hyperoxia on nucleus anomaly, SOD, CAT activities and MDA content in juvenile turbot Scophthalmus maximus[J]. Journal of Shanghai Ocean University, 2011, 20(6): 808-813. |
[23] |
WELKER A F, CAMPOS E G, CARDOSO L A, et al. Role of catalase on the hypoxia/reoxygenation stress in the hypoxia-tolerant Nile tilapia[J]. American Journal of Physiology Regulatory Integrative and Comparative Physiology, 2012, 302(9): R1111-R1118. DOI:10.1152/ajpregu.00243.2011 |
[24] |
邱德全, 周鲜娇, 邱明生. 氨氮胁迫下凡纳滨对虾抗病力和副溶血弧菌噬菌体防病效果研究[J]. 水生生物学报, 2008, 32(4): 455-461. QIU D Q, ZHOU X J, QIU M S. Study on anti-disease ability of Litopenaeus vannamei and the biological control of Vibrio parahaemolyticus bacteriophage under stresses of ammonia nitrogen[J]. Acta Hydrobiologica Sinica, 2008, 32(4): 455-461. DOI:10.3321/j.issn:1000-3207.2008.04.002 |
[25] |
ROMANO N, ZENG C S. Ontogenetic changes in tolerance to acute ammonia exposure and associated gill histological alterations during early juvenile development of the blue swimmer crab, Portunus pelagicus[J]. Aquaculture, 2007, 266(1/4): 246-254. |
[26] |
黄鹤忠, 李义, 宋学宏, 等. 氨氮胁迫对中华绒螯蟹(Eriocheir sinensis)免疫功能的影响[J]. 海洋与湖沼, 2006, 37(3): 198-205. HUANG H Z, LI Y, SONG X H, et al. NH4+-N stress on Mmune function of Eriocheir sinensis[J]. Oceanologia et Limnologia Sinica, 2006, 37(3): 198-205. DOI:10.3321/j.issn:0029-814X.2006.03.002 |
[27] |
洪美玲.水中亚硝酸盐和氨氮对中华绒螯蟹幼体的毒性效应及维生素E的营养调节[D].上海: 华东师范大学, 2007. HONG M L. Toxicity of ambient nitrite and ammonia on Eriocheir sinensis juveniles and nutritional modulation of dietary VE[D]. Shanghai: East China Normal University, 2007. http://cdmd.cnki.com.cn/article/cdmd-10269-2007083144.htm |
[28] |
BAYIR A, SIRKECIOGLU A N, BAYIR M, et al. Metabolic responses to prolonged starvation, food restriction, and refeeding in the brown trout, Salmo trutta: oxidative stress and antioxidant defenses[J]. Comparative Biochemistry and Physiology Part B:Biochemistry and Molecular Biology, 2011, 159(4): 191-196. DOI:10.1016/j.cbpb.2011.04.008 |
[29] |
ROSSI A, CAZENAVE J, BACCHETTA C, et al. Physio-logical and metabolic adjustments of Hoplosternum littorale(Teleostei, Callichthyidae) during starvation[J]. Ecological Indicators, 2015, 56: 161-170. DOI:10.1016/j.ecolind.2015.04.001 |
[30] |
PASCUAL P, PEDRAJAS J R, TORIBIO F, et al. Effect of food deprivation on oxidative stress biomarkers in fish (Sparus aurata)[J]. Chemico-Biological Interactions, 2003, 145(2): 191-199. DOI:10.1016/S0009-2797(03)00002-4 |
[31] |
徐力文, 刘广锋, 王瑞旋, 等. 急性盐度胁迫对军曹鱼稚鱼渗透压调节的影响[J]. 应用生态学报, 2007, 18(7): 1596-1600. XU L W, LIU G F, WANG R X, et al. Effects of abrupt salinity stress on osmoregulation of juvenile Rachycentron canadum[J]. Chinese Journal of Applied Ecology, 2007, 18(7): 1596-1600. DOI:10.3321/j.issn:1001-9332.2007.07.031 |
[32] |
JÜRSS K, BITTORF T, VÖKLER T, et al. Effects of tem-perature, food deprivation and salinity on growth, RNA/DNA ratio and certain enzyme activities in rainbow trout (Salmo gairdneri Richardson)[J]. Comparative Biochemistry and Physiology Part B:Comparative Biochemistry, 1987, 87(2): 241-253. DOI:10.1016/0305-0491(87)90136-2 |
[33] |
WATENPAUGH D E, BEITINGER T L. Nitrite exposure and respiration rates in fathead minnows[J]. Bulletin of Environmental Contamination and Toxicology, 1985, 35(1): 106-111. DOI:10.1007/BF01636487 |
2. Key Laboratory of Freshwater Aquatic Genetic Resources, Ministry of Agriculture and Rural Affairs, Shanghai Ocean University, Shanghai 201306, China;
3. National Demonstration Center for Experimental Fisheries Science Education, Shanghai Ocean University, Shanghai 201306, China