2. 云南省设计院集团勘察院, 昆明 650223
2. Yunnan Design Institute Group Survey Institute, Kunming 650223, China
0 引言
季节冻土地区的路基在冬季冻结,低温冻结时土颗粒间形成毛细孔道,地下水在土体冻结过程中给土体进行水分补给,导致土基中形成冰冻层,而冰冻层在冻结的过程中体积变大[1-2],因此产生了道路路基凸起、路面拥包、柔性路面出现裂缝、刚性路面出现断裂错台或折断等现象[3-6]。春季温度升高,融化的路基土内产生大量的游离态水体,在反复的车辆荷载作用下出现挤泥冒浆现象,致使路基强度降低[7-8]。而且此现象在季节冻土地区路基土反复冻融之下更为严重。要想解决该问题,可在路基内设冷阻层,避免路基土产生冻结。
对道路路基进行保温技术的研究始于20世纪60年代[9]。1969年在美国阿拉斯加的道路中第一次采用聚苯乙烯泡沫(EPS)对道路进行保温处理[10]。加拿大工程专家的研究表明,1 cm厚的EPS相当于14 cm厚的填土保温效果,6 cm厚的EPS可使土体的冻结深度减小50%以上[11]。目前,欧美等国家在道路保温方面积累了大量的成功经验[12]。20世纪70年代中期我国开始在多年冻土地区的青藏高原道路中应用保温技术,研究者[13-14]利用有限元对多年地区的路基温度场进行模拟,表明保温层对多年冻土起到很好的保护作用。目前道路应用的保温材料多用EPS和绝热用挤塑聚苯乙烯泡沫塑料(XPS)两种材料,其中XPS保温效果很好,但其比较脆、受荷载后易断裂、形成应力集中并且伸缩性比较差、造价很高。前人也曾针对季节冻土地区道路的保温技术进行了一些研究,但在保温材料上突破不大。笔者经过前期研究得知粉煤灰土经过多次冻融循环后的强度和动模量都高于粉质黏土,且橡胶颗粒改良粉煤灰土、聚丙烯纤维改良后的粉煤灰土具有高强度的特性;故分别采用粉煤灰土、橡胶颗粒改良粉煤灰土和聚丙烯纤维改良后的粉煤灰土3种材料做冷阻层,利用ANSYS软件热分析原理,模拟冬季路基温度场,对分别设有冷阻层的三维有限元路基模型进行对比、分析,研究各自的阻冷效果,以期待为季节冻土地区路基的保温提供数据参考及理论依据。
1 有限元模型建立 1.1 道路结构路基是沿纵向无限延伸的三维构造物,对路基温度场的研究可取其横断面进行[15]。此外,在季节冻土地区新填筑的道路路基一般选取二灰碎石材料作为路基填料。因此,在建立有限元模型时模拟实际道路情况,自上而下使用5层道路的结构,分别为:厚度为0.17 m、顶面宽度为14.00 m的沥青面层;厚度为0.40 m的二灰碎石层;厚度为0.30 m、分别用3种材料做的冷阻层;厚度为1.23 m的路基土;土基。道路边坡坡度为1:1.5(图 1)。冷阻层材料分别为粉煤灰土、橡胶颗粒改良粉煤灰土及聚丙烯纤维改良粉煤灰土。
![]() |
图 1 道路横断面图 Figure 1 Cross section drawing of the road |
|
为使计算更加准确,考虑到下伏土层和临近土层的影响,将ANSYS计算的三维有限元模型设定如下:计算宽度取边坡以外10.0 m,共计30.3 m;路基长度为20.0 m;计算深度取12.1 m;采用最大尺寸为0.5 m的自由网格;热分析采用solid 70八节点实体单元(图 2)。
![]() |
图 2 ANSYS模型示意图 Figure 2 ANSYS model figure |
|
利用导热系数和比热试验的测试仪及其他常规仪器,通过室内试验的方法得到沥青面层、二灰碎石、粉质黏土、粉煤灰土、橡胶颗粒改良粉煤灰土、聚丙烯纤维改良粉煤灰土6种材料的厚度、导热系数、比热容和密度(表 1)。
材料名称 | 厚度/m | 导热系数/(W/(m·K)) | 比热容(J/(g·℃)) | 密度/(g/cm3) |
沥青面层 | 0.17 | 1.05 | 1.17 | 2.3~2.5 |
二灰碎石 | 0.40 | 1.51 | 1.00 | 2.21 |
粉质黏土 | 1.23 | 1.16 | 1.58 | 1.52 |
粉煤灰土 | 0.30 | 0.28 | 0.78 | 1.93 |
橡胶颗粒改良粉煤灰土 | 0.30 | 0.22 | 0.62 | 1.92 |
聚丙烯纤维改良粉煤灰土 | 0.30 | 0.26 | 0.62 | 1.92 |
1) 初始条件的确定
路基温度场的边界条件比较复杂,因此在计算前要对边界条件进行约定。为计算季节冻土地区路基冷阻层温度场,本文采用长春冬季的温度场变化特性。由于长春从10月开始出现负温,故计算的开始时间定为10月1日。因地面温度与大气温度较接近,因此地面温度采用9月的平均大气温度15.5 ℃[16]。长春冬季最大冻深约1.5 m,因此土基在地面以下3.0 m处的温度取地面温度15.5 ℃。通过上述约定,将计算路基模型的边界温度条件加以设定。
2) 对流换热边界条件
材料的对流换热与材料本身导热系数和材料内大气流体温度有关。材料的导热系数采用室内试验测得数据,大气流体温度取日平均温度。日平均温度t与最热月平均气温、最冷月平均气温、区域参数等因素有关,计算公式[17]为
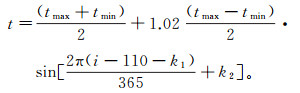
式中:tmax为最热月平均气温;tmin为最冷月平均气温;i为日序,计算起始日期i=1;k1、k2为区域参数。对于处于东北内陆的长春地区:当i≤180时,k1=0,
本文的计算周期为4月,计算的起始时间为10月1日,终止时间为次年的1月31日,i取值为1~123。由文献[17]查得tmax=23.1 ℃,tmin=-15.1 ℃,由式(1)计算得到长春地区从10月1日至次年的1月31日约120 d内的温度变化曲线(图 3)。
![]() |
图 3 温度随时间变化图 Figure 3 Temperature variation over time |
|
由图 3可知,在计算周期内大气的日平均温度呈先下降后上升趋势,在12月中下旬温度达到最低点。
1.2.3 模型求解路基的温度场是三维非稳态,随着大气温度变化,边界条件也随之而变,路基内部温度也在变化。在对模型进行施加温度-时间变化曲线的荷载时,由于前期计算的是日平均温度,故将荷载步步长定义为1 d,以对模型进行非线性时程分析[18]。
2 计算结果分析研究材料的冷阻效果,需经过一段时间的负温作用分析路基温度场的变化情况。通过有限元软件计算得到3种不同冷阻层材料的路基温度场等温线和云图、路基内部和土基的温度随时间变化情况、温度随深度变化情况和温度梯度。
2.1 粉煤灰土的冷阻效果将粉煤灰土设定为冷阻层材料的道路路基在经过120 d的负温作用后,有限元软件分析得到路基温度场云图、道路中线不同深度土层温度随时间的变化曲线和第120天路基温度梯度(图 4)。
![]() |
图 4 粉煤灰土路基温度场云图(a)、温度随时间的变化曲线(b)及第120天的温度梯度(c) Figure 4 Cloud picture of temperature field(a), variation curve of temperature with time(b) and temperature gradient of 120th day(c) of flyash-lime soil subgrade |
|
由图 4可以看出:粉煤灰土作为冷阻层的路基经过120 d的负温作用,路基温度由上至下逐渐升高,土基中温度最高值为14.493 ℃(图 4a);路基各层的温度都低于起始温度,道路中线以下0.00、0.17和0.57 m层面的温度先随时间的增长而降低,当达到最低点后开始回温,而地面下1.28 m以下的土层始终处于零上温度(图 4b);路基最大冻深(0 ℃对应的深度)为1.32 m,温度梯度最低值为-17.606 ℃/m(图 4c)。
2.2 橡胶颗粒改良粉煤灰土的冷阻效果将橡胶颗粒改良粉煤灰土设定为冷阻层材料的道路路基在经过120 d的负温作用后,有限元软件分析得到路基温度场云图、道路中线不同深度土层温度随时间的变化曲线和第120天路基温度梯度(图 5)。
![]() |
图 5 橡胶颗粒改良粉煤灰土路基温度场云图(a)、温度随时间的变化曲线(b)及第120天的温度梯度(c) Figure 5 Cloud picture of temperature field(a), variation curve of temperature with time(b) and temperature gradient of 120th day(c) of fly ash-lime soil subgrade modified by rubber particles |
|
图 5显示:橡胶颗粒改良粉煤灰土作为冷阻层的路基经过120 d的负温作用,路基温度由上至下逐渐升高,土基中温度最高值为14.611 ℃(图 5a);路基各层的温度都低于起始温度,道路中线以下0.00、0.17和0.57 m层面的温度先随时间的增长而降低,当达到最低点后开始回温,地面下0.87 m以下的土层均出现零下温度,地面以下1.28、1.69和2.10 m的土层始终处于零上温度(图 5b);路基的最大冻深为0.94 m,温度梯度最低值为-23.563 ℃/m(图 5c)。
2.3 聚丙烯纤维改良粉煤灰土的冷阻效果将聚丙烯纤维改良粉煤灰土设定为冷阻层材料的道路路基在经过120 d的负温作用后,有限元软件分析得到路基温度场云图、道路中线不同深度土层温度随时间的变化曲线和第120天路基温度梯度(图 6)。
![]() |
图 6 聚丙烯纤维改良粉煤灰土温度场云图(a)、温度随时间的变化曲线(b)及第120天的温度梯度(c) Figure 6 Cloud picture of temperature field(a), variation curve of temperature with time(b) and temperature gradient of 120th day (c) of polypropylene fiber modified fly ash soil |
|
图 6显示:聚丙烯纤维改良粉煤灰土作为冷阻层的路基经过120 d的负温作用,路基温度由上至下逐渐升高,土基中温度值最最高值为14.528 ℃(图 6a);路基各层的温度都低于起始温度,道路中线以下0.00、0.17和0.57 m层面的温度先随时间的增长而降低,当达到最低点后开始回温,道路中线下0.87 m土层在第74天后温度处于零下,道路中线下1.28 m以下的土层始终处于零上温度(图 6b);路基的最大冻深为1.20 m,温度梯度最低值为-19.557 ℃/m(图 6c)。
2.4 3种冷阻材料的冷阻效果对比有限元软件计算3种冷阻层材料的层顶温度-时间关系曲线和层底温度-时间关系曲线见图 7,同时,对第30天、第60天、第90天和第120天的冷阻层层底温度进行提取(表 2),对3种材料的道路的最大冻深及温度梯度加以对比(表 3)。
![]() |
a.冷阻层顶面;b.冷阻层底面 图 7 3种冷阻层顶、底面温度随时间的变化曲线 Figure 7 Change curve of the top and bottom temperature of three kinds of cold resistive layer with time |
|
℃ | |||
i | 粉煤灰土 | 橡胶颗粒改良粉煤灰土 | 聚丙烯纤维改良粉煤灰土 |
30 | 6.74 | 7.99 | 7.11 |
60 | 1.17 | 2.98 | 1.75 |
90 | -2.16 | -0.15 | -1.48 |
120 | -1.99 | -0.28 | -1.39 |
材料 | 最大冻深/m | 温度梯度最低值/(℃/m) |
粉煤灰土 | 1.32 | -17.606 |
橡胶颗粒改良粉煤灰土 | 0.94 | -23.563 |
聚丙烯纤维改良粉煤灰土 | 1.20 | -19.557 |
由图 7、表 2和表 3可以看出:在设有3种冷阻材料的路基结构中,3种材料层顶面的温度相同,但橡胶颗粒改良粉煤灰土冷阻层的底面温度高于其他2种材料;采用橡胶颗粒改良粉煤灰土为冷阻层的道路最大冻深为0.94 m,比粉煤灰土的最大冻深小0.38 m,比聚丙烯纤维改良粉煤灰土的最大冻深小0.26 m;橡胶颗粒改良粉煤灰土的温度梯度最低值为-23.563℃/m,粉煤灰土的温度梯度最低值为-17.606℃/m,聚丙烯纤维改良粉煤灰土的温度梯度最低值为-19.557℃/m。说明采用橡胶颗粒改良粉煤灰土的冷阻效果最好。
2.5 最小冷阻层厚度的确定橡胶颗粒改良粉煤灰土的冷阻效果最佳,但为了降低工程造价,在确保土基不冻的前提下,应确定最小的橡胶颗粒改良粉煤灰土铺筑厚度。有限元模型建立时与上述模型相同,只是改变橡胶颗粒改良粉煤灰土冷阻层的厚度,利用上述的有限元软件的分析原理及边界条件分析得到120 d内冷阻层底层的最低温度(表 4、图 8)。
序号 | 厚度/m | 最低温度/℃ | 日序 |
1 | 0.30 | -0.628 | 106 |
2 | 0.31 | -0.416 | 106 |
3 | 0.32 | -0.210 | 107 |
4 | 0.33 | -0.008 | 107 |
5 | 0.34 | 0.189 | 108 |
6 | 0.35 | 2.414 | 118 |
![]() |
图 8 路基土顶层温度随橡胶颗粒改良粉煤灰土冷阻层厚度的变化 Figure 8 Temperature variation of the subgrade top layer with the depth of the cold resistance layer of fly ash-lime soil subgrade modified by rubber particles |
|
由表 4和图 8可以看出:确保路基土处于零上温度不冻结状态的最小冷阻层(橡胶颗粒改良粉煤灰土)摊铺厚度为0.33 m。
3 结论1) 设有3种材料冷阻层路基的温度由上至下温度逐渐升高,其中冷阻层为橡胶颗粒改良粉煤灰土的土基中温度升高值最大,达到14.611 ℃;路基各层的温度经过120 d后都低于起始温度,设有橡胶颗粒改良粉煤灰土冷阻层的道路中线0.87 m以下的土层始终处于零上温度。
2) 计算周期内3种材料层的顶面温度相同,但设有橡胶颗粒改良粉煤灰土冷阻层的层底面的温度高于其他两种材料;采用橡胶颗粒改良粉煤灰土为冷阻层的道路冻深为0.94 m,比粉煤灰土的最大冻深小0.38 m,比聚丙烯纤维改良粉煤灰土的最大冻深小0.26 m;橡胶颗粒改良粉煤灰土的温度梯度最低值为-23.563 ℃/m,粉煤灰土的温度梯度最低值为-17.606 ℃/m,聚丙烯纤维改良粉煤灰土的温度梯度最低值为-19.557℃/m。
3) 橡胶颗粒改良粉煤灰土冷阻效果最佳,确保路基土处于零上温度不冻结状态的最小冷阻层摊铺厚度为0.33 m。
[1] | Qin Yinghong. Theory and Procedure for Measuring the Albedo of a Roadway Embankment[J]. Cold Regions Science and Technology, 2016, 126: 30-35. DOI:10.1016/j.coldregions.2016.03.005 |
[2] |
王锐, 程培峰, 韩春鹏, 等. 高纬度多年冻土区路基工后沉降变形[J].
长安大学学报(自然科学版), 2017, 37(1): 43-49, 75.
Wang Rui, Cheng Peifeng, Han Chunpeng, et al. Roadbed Settlement Deformation After Construction in Permafrost Regions of High Altitude[J]. Journal of Chang'an University(Natural Science Edition), 2017, 37(1): 43-49, 75. |
[3] | Gruber S. Review Article:Inferring Permafrost and Permafrost Thaw in the Mountains of the Hindu Kush Himalaya Region[J]. The Cryosphere, 2017, 11: 88-99. |
[4] | Fan Yu. Crack Formation of a Highway Embankment Installed with Two-Phase Closed Thermosyphons in Permafrost Regions:Field Experiment and Geothermal Modelling[J]. Applied Thermal Engineering, 2017, 115: 670-681. DOI:10.1016/j.applthermaleng.2017.01.001 |
[5] | Zheng Mingyan. Modeling and Mitigation of Excessive Dynamic Responses of Wind Turbines Founded in Warm Permafrost[J]. Engineering Structures, 2017, 148: 36-46. DOI:10.1016/j.engstruct.2017.06.037 |
[6] |
李金平, 张娟, 陈建兵, 等. 高寒冻土区路基变形演化规律与破坏特征[J].
交通运输工程学报, 2016, 16(4): 78-87.
Li Jinping, Zhang Juan, Chen Jianbing, et al. Evolution Laws and Failure Characteristics of Subgrade Deformation in Alpine Permafrost Region[J]. Journal of Traffic and Transportation Engineering, 2016, 16(4): 78-87. |
[7] | Tai Bowen. Thermal Characteristics and Declining Per-mafrost Table Beneath Three Cooling Embankments in Warm Permafrost Regions[J]. Applied Thermal Engineering, 2017, 123: 435-447. DOI:10.1016/j.applthermaleng.2017.05.031 |
[8] | Jonathan D. Review of Effectiveness and Costs of Stra-tegies to Improve Roadbed Stability in Permafrost Regions[J]. Regehr Journal of Cold Regions Engineering, 2013, 27(3): 109-131. DOI:10.1061/(ASCE)CR.1943-5495.0000054 |
[9] |
盛煌, 张鲁新, 杨成松, 等. 保温处理措施在多年冻土区道路工程中的应用[J].
冰川冻土, 2002, 24(5): 618-622.
Sheng Huang, Zhang Luxin, Yang Chengsong, et al. Application of Thermal-Insulation Treatment to Roadway Engineering in Permafrost Regions[J]. Journal of Glaciology and Geocryology, 2002, 24(5): 618-622. |
[10] |
温智, 盛煌, 马巍. EPS保温板在青藏铁路中应用的适用性评价数值模拟[J].
铁道学报, 2005, 27(3): 91-96.
Wen Zhi, Sheng Huang, Ma Wei, et al. Numerical Simulation on Application of EPS in Embankment Engineering of Qinghai-Tibet Railway[J]. Jouranl of the China Railway Society, 2005, 27(3): 91-96. |
[11] |
王曼, 刘建军. 道路工程中XPS保温板防冻胀厚度的研究[J].
石河子大学学报(自然科学版), 2014, 32(1): 123-127.
Wang Man, Liu Jianjun. Study on Anti-Frost Thickness of Insulation in Road Engineering[J]. Journal of Shihezi University (Natural Science), 2014, 32(1): 123-127. |
[12] |
刘素芳, 石磊, 郑方园, 等. 聚苯乙烯泡沫塑料在建筑设计中的应用[J].
塑料工业, 2016, 44(12): 95-98.
Liu Sufang, Shi Lei, Zheng Fangyuan, et al. The Application of Polystyrene Foam in Architectural Design[J]. China Plastice Industry, 2016, 44(12): 95-98. DOI:10.3969/j.issn.1005-5770.2016.12.022 |
[13] |
李杨, 王清, 王坛华, 等. 冻土水热耦合模型数值求解及结果检验[J].
吉林大学学报(地球科学版), 2015, 45(1): 207-213.
Li Yang, Wang Qing, Wang Tanhua, et al. Numerical Solution and Test of Results for a Hydrothermal Coupled Model About Frozen Soil[J]. Journal of Jilin University (Earth Science Edition), 2015, 45(1): 207-213. |
[14] |
马勤国, 赖远明, 吴道勇, 等. 多年冻土区高等级公路路基温度场研究[J].
中南大学学报(自然科学版), 2016, 32(7): 2415-2423.
Ma Qinguo, Lai Yuanming, Wu Daoyong, et al. Analysis of Temperature Field of High Grade Highway Embankment in Permafrost Regions[J]. Journal of Central South University (Science and Technology), 2016, 32(7): 2415-2423. DOI:10.11817/j.issn.1672-7207.2016.07.002 |
[15] |
刘海苹, 丁琳, 杨扬, 等. 路基高度对高纬度多年冻土区路基温度场的影响[J].
黑龙江大学工程学报, 2017, 8(1): 25-29.
Liu Haiping, Ding Lin, Yang Yang, et al. Influence of Subgrade Height in Subgrade Temperature Field on High Latitude Permafrost Regions[J]. Journal of Engineering of Heilongjiang University, 2017, 8(1): 25-29. |
[16] |
邰博文, 刘建坤, 房建宏, 等. 高温冻土区高速公路特殊结构路基地温分布特征及降温效果分析[J].
岩石长安大学学报(自然科学版), 2017, 36(增刊1): 3696-3704.
Tai Bowen, Liu Jiankun, Fang Jianhong, et al. Analysis on Distribution Characteristics of Ground Temperature and Cooling Effect of Special-Structured Subgrade of the Expressway in Warm Permafrost Region[J]. Chinese Journal of Rock Mechanics and Engineering, 2017, 36(Sup.1): 3696-3704. |
[17] |
姚运生, 王艳玲. 我国北方逐日平均气温的气候模拟[J].
中国农业气象, 2004, 25(3): 5-7.
Yao Yunsheng, Wang Yanling. Climate Simulation on Daily Mean Temperature in Norehern China[J]. Chinese Journal of Agrometeorology, 2004, 25(3): 5-7. |
[18] |
程培峰, 王锐, 韩春鹏, 等. 高纬度多年冻土区路基工后温度变化规律研究[J].
公路工程, 2017, 42(5): 12-17, 31.
Cheng Peifeng, Wang Rui, Han Chunpeng, et al. Study on the Permafrost Roadbed the Temperature Change Rule After Constructed at the High Altitude[J]. Highway Engineering, 2017, 42(5): 12-17, 31. |