1 引言
过去1000年来的气候变化是衔接过去代用指标序列和现代器测资料记录的关键时段,也是人类活动影响不断加剧的时期。在这一时期,充足的历史文献资料记载了人类社会发展的兴衰,而高分辨率气候记录的重建为研究人类社会与气候变化之间的关联提供了有利条件[1, 2, 3, 4]。因此,研究过去1000年气候变化的可能驱动机制对深入认识气候系统的演化规律和在综合考虑自然因子及人为因子影响下实现未来气候的准确预测有着重要意义[5, 6, 7, 8, 9]。季风作为快速演化的物理系统,对海-气-冰等边界条件变化的响应尤为敏感[10],并通过大气/洋流作用紧密联系着南北半球气候信号[11]。中低纬度典型季风区的季风演化作为全球气候变化的重要组成部分是深入了解全球气候变化机制的重要渠道之一[12]。随着科技的不断进步及精密仪器的出现,季风区内的石笋研究取得了巨大的成果[1, 11, 13, 14, 15, 16, 17, 18],尤其是世界上最典型的亚洲季风区[19, 20, 21, 22]。因自身定年准确、 封闭性好和分辨率高等优点,洞穴石笋沉积物成了研究中、 低纬度季风演变的重要代用指标[23, 24, 25, 26, 27]。本文选取了采自于印度中部地区Jhumar洞的一支高分辨率石笋作为印度夏季风的代用记录[28],尝试以此为切入点反演并量化分析近千年印度夏季风百年尺度变化的可能驱动机制,为深入认识印度夏季风演化规律、 预测未来气候变化提供参考依据。
结合传统统计模型和动力模型的优点,黄建平和衣育红[29]通过差分近似导数建立了自治的定步长非线性动力反演模型,它可以充分利用观测数据探求系统可能存在的动力机制; 林振山和史芳斌[30]通过反演模型,利用天津1932年1月到1984年12月的气压、 温度和降水资料反演出局地气候的温度-气压-降水动力方程,为天津局地气候研究提供了重要理论依据,也为深入研究其他局地气候系统提供了新思路; 罗虎明等[31]在前人研究基础上通过可变步长的非自治反演非线性动力模型探讨了东亚季风的驱动机制,为季风动力反演提供了可借鉴经验。虽然这种方法对季风驱动机制的反演存在一定的不确定性,我们所得到的结果也许只是古季风复杂驱动机制中的一种,但是在我们对古季风驱动机制一直处于定性描述及缺乏量化分析的现阶段,通过反演得到一种可能存在的古季风驱动机制,对于我们深入了解并研究古季风驱动机制无疑是具有可借鉴意义的。
由于太阳辐射是地球外部能量的惟一来源,通常被认为是影响地球气候变化的最重要因素[32, 33, 34, 35, 36],也有众多气候记录表明太阳活动周期和气候变化之间存在联系[11, 14, 32, 37],用数学模型模拟近1000年温度特征时,也往往直接将太阳活动作为主要强迫因子[38, 39],但是太阳辐射能量变化在过去几十年甚至几千年里都是很小的(1~2mW/m2)[40],使人质疑如此小幅度的太阳辐射变化是如何驱动地球历史中一次又一次的巨大气候突变事件,从而影响气候变化的[41, 42]?一些学者认为是海/气循环系统将微小的太阳变化信号放大[43],其中北大西洋的放大机制假说颇受赞同。大量研究表明全新世期间亚洲季风突变事件和北大西洋冷事件存在密切联系[11, 44, 45, 46, 47, 48, 49, 50, 51],Asmerom等[52]通过对美国西南部石笋记录的研究发现增强的太阳辐射与当地的干旱事件相对应,而这种对应关系恰恰与季风相反,从而提出太阳辐射量变化通过赤道太平洋沃克环流、 PDO以及ENSO等控制着季风和美国西南部的气候变化的观点。火山喷发也是不容忽视的一个影响因素[53, 54, 55, 56]。而吴国雄和张永生[57]认为青藏高原的机械强迫作用和热力作用是亚洲季风爆发的诱因。IPCC第四次评估报告指出[58],20世纪中期以来主要观测到的全球气温上升有大于90 % 的可能性是由于人类排放的温室气体浓度增加所引起的。然而,由于地质资料和技术方法的限制,至今我们对印度夏季风降水机理都没有一个全面清晰的认识,统计-动力反演就是在有限资料的基础上,通过数理方法找出印度夏季风和其他驱动因子之间的可能联系,为认识印度夏季风驱动机制提供参考依据。
2 方法和数据 2.1 非线性动力反演模型非线性动力反演模型中,设非线性系统的状态变量x1随时间变化的动力方程为:
如果f[t, x1(t), x2(t),…, xn(t)]可以分为k1部分,即:
方程(2)可以写成矩阵形式:
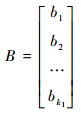
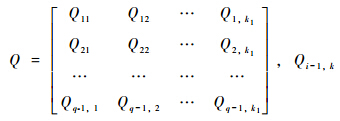
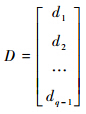

在方程(4)中,只有B是未知的,也是线性的。要求得矩阵B,可以运用矩阵的最小二乘估计及反演理论来求解矩阵B[29, 30]。得到系数B后,可进一步分析f中各项的相对贡献率Wk:
根据各项贡献率大小剔除对系统演变无用或作用很小的项,最后得到所需方程或方程组。如要提高精度,可在剔除无关项的基础上再反演一次。统计-动力反演的建模过程不仅摒弃了复杂的物理要素筛选,而且也不需要人为决定动力方程的形式,所以建立起来的模型可以说是几乎没有主观因素影响。其次,可以很直观的找出系统演化有关的动力因子(系数为正)和阻力因子(系数为负)。再者,各项相对贡献率大小的确定使得量化分析其对系统演化作用的大小成为可能,为量化分析季风动力机制提供了新思路。最后,可以预测系统演化趋势。
2.2 数据Jhumar洞位于印度中央邦东南端的杰格德尔布尔(Jagdalpur)城镇附近(18°52′N, 81°52′E;600m a .s .l.),降水分布不均,年降水量主要受季风降水控制,集中在7~9月,约占据当地年降水量的70 % ~80 % [28]。此外,Jhumar洞石笋JHU-1的 δ 18 O值曲线[28]与Dandak洞[59]石笋 δ 18 O值曲线有很好的重叠,说明Jhumar洞石笋 δ 18 O值曲线具有一定的代表性。较其他印度夏季风代用资料而言,Jhumar洞石笋具有时间尺度长、 分辨率高、 地理位置居中等优点,因此本文选取了1年分辨率的Jhumar洞石笋 δ 18 O作为印度夏季风代用资料对印度夏季风进行反演,定量分析其百年尺度上的驱动机制。石笋的 δ 18 O值被认为储存了大量的气候综合信息,尤其是温度和降水,而在季风区这个指标往往受到季风强度的影响,因此国内外大多学者认为石笋的 δ 18 O值负偏表明季风增强,降水增多; 相反,石笋的 δ 18 O值正偏表明季风减弱,降水减少[11, 13, 26]。具体代用资料数据详见 表 1。
![]() |
表 1 印度夏季风可能的影响因子代用资料数据 Table 1 Proxies of Indian summer monsoon driving factors |
表 1所列代用资料数据均通过3次样条函数以1年步长插值到1000~1950A .D . 时段,并作标准化处理。青藏高原普若岗日冰芯 δ 18 O数值越大,表明温度越高; 南极Law Dome冰芯CO2、 CH4和N2O数值越大,表明温度越高。
3 模型及其反演结果设印度夏季风驱动机制的动力模型为:
在方程(6)中,表示t时刻的季风强度变率,a1,a2,…,a104为未知系数,m,s,…,r分别为 表 1中各可能的影响因子,交叉项表示影响因子的相互作用。从动力学角度来讲,多项式中每一项都对整个系统演化起到一定的作用: 系数为正,则表示起动力作用; 系数为负,则表示起负反馈稳定作用。
将表 1中石笋 δ 18 O、 太阳TSI、 ENSO、 PDO、 温室气体浓度、 北大西洋海表温、 阿拉伯海海表温度、 青藏高原冰芯 δ 18 O、 GISPⅡ冰芯、 James Rose冰芯和火山灰硫酸盐数据在 ti(i=1000 1001,…,1950)时刻的值作为方程(6)的一系列数值解,通过反演模型(6)得到各项系数值及相应各项的贡献率。再取1000A .D . 的石笋 δ 18 O数值作为初值,设步长为1年,用四阶Runge-Kutta定步长算法计算出方程(6)的数值解[70]。反演结果与真实值对比如 图 1所示,其相关系数为0.8597。
![]() |
图 1 1000~1950A .D . 石笋 δ 18 O的模拟值(红色)与真实值(黑色)对比 Fig. 1 Comparison between the inversion result (red) and the observation data (black) of the δ 18 O of stalagmite between 1000A .D . and 1950A .D . |
由于方程略显繁冗,因此将贡献率小于0.0001设置为剔除条件,剩余的90项的累计贡献率为99.94 % ,剔除掉贡献率较小的14项后重新建立反演方程如下:
方程(7)用原数据按前面步骤重新反演,各项系数及贡献率见 表 2(按贡献率由大到小排序,粗体黑为驱动项,非粗体为稳定项)。
![]() |
表 2 方程(7 )反演结果 Table 2 Inversion results of the equation(7) |
依然取1000A .D . 的Jhumar洞石笋 δ 18 O数值作为初值,步长1年,用四阶Runge-Kutta定步长算法计算出方程(7)的数值解。反演结果与真实值对比如 图 2所示,得到的反演值与真实值的相关系数为0.8527,相对104项的反演结果而言几乎没有差别,说明方程(7)可以作为近千年印度夏季风的驱动方程,并可以很好地反映印度夏季风的驱动机制。
![]() |
图 2 1000~1950A .D . 石笋 δ 18 O的模拟值(剔除14项后)(红色)与真实值(黑色)对比 Fig. 2 Comparison between the inversion result (obtain from an inversion equation which is taken 14 terms out)(red) and the observation data (black) of the δ 18 O of stalagmite between 1000A .D . and 1950A .D . |
方程(6)右边的104个单项式中贡献率大于1 % 的有24项,其贡献率之和约为85.76 % ,说明这24个单项式是印度夏季风的24个主要的驱动和稳定因子,其中系数为正的是驱动项,起驱动作用; 系数为负的是稳定项,起稳定作用。此外,这24项中仅有4个一次项,累计贡献率为16.13 % ,其余20个均为二次项(包括平方项和交叉项),累计贡献率为69.63 % ,说明印度夏季风系统是一个复杂的非线性系统,其驱动机制并非简单的线性驱动。
方程(7)中保留下的11个线性项中,北大西洋海表温、 甲烷浓度、 南极温度、 北极温度、 太平洋涛动、 ENSO和太阳辐照度项的系数为正,表明它们对近千年印度夏季风演化起到驱动作用,说明印度夏季风不仅受到太阳和两极冰量的影响,中高纬度洋流也是印度夏季风的控制因素之一。
自工业革命以来,全球环境面临着气候变暖、 酸雨危害等一系列重大全球性环境问题的考验,其中温室气体含量在工业革命前后显示出的显著不同特征成为最受瞩目的科学和社会问题之一,引起了全世界的重视。IPCC第五次评估报告的第一工作组报告《气候变化2013: 自然物理基础》[58, 71]也指出:“气候系统暖化是毋庸置疑的事实”。Sarthi等[72]研究了气候变暖情况下印度夏季风特征的可能变化,指出温室效应对季风降水的影响比海陆热力差异更为突出。IPCC[58]也指出全球变暖对季风降水有影响,且未来区域气候变化很可能主要归因于温室效应。鉴于此,本文将1000~1950A .D . 分为1000~1799A .D . 和1800~1950A .D . 两个时段分别反演,以便更好的分析不同气候背景下印度夏季风的驱动机制。选取1800A .D . 不是1850A .D . 作为分界点是受到了反演方法对时间序列长度需求的限制。反演结果如 图 3和 图 4所示,相关系数分别为0.9249和0.9399。方程右边各项系数和贡献率分别见 表 3和 表 4(按贡献率由大到小排序,粗体为驱动项,非粗体为稳定项)。
![]() |
图 3 1000~1799A .D . 石笋 δ 18 O的模拟值(红色)与真实值(黑色)对比 Fig. 3 Comparison between the inversion result(red)and the observation data (black) of the δ 18 O of stalagmite between 1000A .D . and 1799A .D . |
![]() |
图 4 1800~1950A .D . 石笋 δ 18 O的模拟值(红色)与真实值(黑色)对比 Fig. 4 Comparison between the inversion result(red)and the observation data(black)of the δ 18 O of stalagmite between 1800A .D . and 1950 A .D. |
![]() |
表 3 1000~1799A .D . 时段方程(6 )反演结果 Table 3 Inversion results of the equation (6) between 1000A .D . and 1799A .D . |
![]() |
表 4 1800~1950A .D . 时段方程(6 )反演结果 Table 4 Inversion results of the equation (6) between 1800A .D . and 1950A .D . |
如 表 3和 表 4所示,贡献率大于1 % 的二次项累计贡献率分别为55.72 % 和88.14 % ,而贡献率大于1 % 的线性项的累计贡献率分别只有27.12 % 和2.30 % ,表明印度夏季风系统主要受到复杂的非线性力控制。其中系数为正的项的中线性项累计贡献率也小于非线性项,也说明印度夏季风变化并非只受简单的单因子驱动,而是各种因素相互作用,共同驱动印度夏季风的变化,这也正说明了地球系统内部各种作用力的相互作用是不容忽视的。
表 3显示系数为正、 贡献率最高的项是北大西洋海表温的线性项,其次有N2O和阿拉伯海表温交叉项、 太阳辐照度和CO2交叉项、 N2O平方项、 ENSO和南极温度的交叉项、 CO2的平方项、 ENSO和CO2的交叉项、 ENSO和N2O的交叉项、 ENSO的线性项及北大西洋海表温的平方项等,说明这些因子及其相互作用是1000~1800A .D . 时段印度夏季风变化的最主要驱动力。由此发现,在近千年印度夏季风演化过程中,北大西洋、 温室气体和ENSO起到了不可小觑的作用,尤其是北大西洋。 表 4显示的系数为正的项中贡献率最高的是甲烷的平方项,其次为CO2和N2O的交叉项、 CO2的平方项、 甲烷和北大西洋海表温的交叉项、 太阳辐射度和CO2的交叉项、 ENSO和甲烷的交叉项、 CO2和北极温度的交叉项以及北大西洋海表温的平方项等,说明工业革命之后温室气体代替了北大西洋海表温成为了最重要的印度夏季风变化的影响因素,但北大西洋、 太阳辐射度、 ENSO和北极温度的作用也不可忽视,温室气体并非单独影响印度夏季风变化,而是和这些因子相互作用,共同影响印度夏季风的变化。
表 3中系数为负、 贡献率最高的项是季风背景值,其次是CO2和北极温度的交叉项,贡献率分别为9.35 % 和6.36 % ,表 4中系数为负、 贡献率最高的项是甲烷和CO2的交叉项,其次是CO2和北大西洋海表温的交叉项,贡献率分别为39.93 % 和7.90 % ,说明季风背景值以及甲烷和CO2的相互作用分别是1800A .D . 前后印度夏季风系统的主要稳定因素。在所有反演结果中,季风背景值的线性项无一例外都是负系数,可能是因为季风有保持自身状态不变的惯性。
比较 表 3和 表 4中贡献率大于1 % 的各项,很容易发现 表 4中除北大西洋海表温度的平方项和南极温度线性项这两项外,其余都是温室气体的平方项和温室气体与其他因子的交叉项,而 表 3中的项则更为复杂,说明工业革命以来温室气体浓度的急剧增加对印度夏季风变化的驱动机制起到了一定程度的改变作用。另外,从 表 4中可以看到甲烷和N2O的线性项系数都为正,而甲烷和N2O的交叉项系数为负,这揭示了温室气体在季风动力系统中两个截然不同的动力学方面: 甲烷与N2O单独可以起驱动作用,而它们之间的非线性相互作用还可以起负反馈稳定作用。这也从侧面表明了印度夏季风是一个复杂的非线性系统,其驱动机制也是各种复杂的非线性作用力共同作用的结果,有着牵一发而动全身的效果。
4 讨论与结论古气候学定量研究起步于第四纪冰期的重建,重点是冷热变化和高纬过程。地质历史上“暖室期”要比“冰期”长得多,季风作为暖室期中气候周期性表现的主要形式,在地球历史上比冰量更为普遍。因此,全球季风等低纬过程的研究,能够揭示地球表层系统演变的一些基本机理[12]。印度夏季风系统是全球最典型的亚洲季风区的一个不可或缺的子系统,它带来的季风降水占东南亚年降水量的将近80 % ,为该地区的农业生产提供了至关重要的水源,从而为近四分之一的世界人口的提供了生产和生活保障[28]。研究并预测印度夏季风在长时间尺度上的变化和未来趋势,不仅是古气候学的当务之急,也能为社会生产生活提供可借鉴科学依据。
本文经反演定量分析发现,印度夏季风系统并非单因子或多因子驱动的简单线性系统,而是多因子相互交叉共同驱动的复杂非线性动力系统。南极温度、 北极温度、 太平洋涛动、 ENSO和太阳辐照度这些单因子对印度夏季风系统的驱动贡献虽然不大,但也不容忽视,也许它们中任意一项的变化都会直接影响印度夏季风强度。其中,太平洋涛动是一种存在于太平洋年-年代际尺度上的气候变率强信号,是太平洋海气环流的重要指标之一[73],近500年的PDO指数与董哥洞石笋 δ 18 O记录的对比显示出相似的年代际振荡特征[74],本文此处印证了印度夏季风和太平洋涛动之间的遥相关关系,由于PDO项系数为正,表明这种关系很有可能是驱动关系。本研究的反演结果都显示火山喷发并非主要的印度夏季风变化的影响因子,表 2显示火山硫酸盐的平方项系数为负,表 3中火山硫酸盐线性项和二次项的系数均为负,说明火山硫酸盐在印度夏季风变化中起稳定作用,而在 表 4中发现火山硫酸盐的线性项和二次项系数均为正,说明在工业革命后期,由于温室气体的影响,火山硫酸盐对印度夏季风起到了驱动作用,但是过程还不得而知。
在工业革命以前,北大西洋海表温、 温室气体(N2O、 CO2)和阿拉伯海表温、 太阳辐照度、 ENSO等的相互作用等是印度夏季风的主要驱动力。尤其是北大西洋海表温,是最主要的驱动力。Zhang和Delworth[75]也通过模拟实验表明北大西洋THC的变化会影响印度夏季风的强弱。而在工业革命以后,由于温室气体浓度的急剧增加,温室气体(CH4、 CO2和N2O)及其与北大西洋海表温、 太阳辐照度、 ENSO及北极温度之间的相互作用成为了印度夏季风的主要驱动力。而季风背景值、 甲烷和CO2的相互作用则是工业革命前后印度夏季风最主要的稳定作用力。工业革命前后印度夏季风主要影响因子的不同表明在气候显著变暖的环境下印度夏季风的驱动机制也随之发生了改变,因此在预测未来气候变化时气候变暖的背景是不可忽视的。
本文运用非线性动力反演方法量化探索研究了印度夏季风的主要影响因子,并对其可能存在的一种驱动机制进行了探讨,但未能对系统响应于各个驱动因子变化的敏感度进行深入分析,这将是今后工作的研究重点。
致谢 感谢审稿专家建设性的修改意见。
1 | Zhang P, Cheng H, Edwards R L et al. A test of climate, sun, and culture relationships from an 1810-year Chinese cave record. Science, 2008,322(5903):940~942 |
2 | 郑景云, 葛全胜, 郝志新等, 历史文献中的气象记录与气候变化定量重建方法. 第四纪研究, 2014,34(6):1186~1196 Zheng Jingyun, Ge Quansheng, Hao Zhixin et al. Paleoclimatology proxy recorded in historical documents and method for reconstruction on climate change. Quaternary Sciences, 2014,34(6):1186~1196 |
3 | 张德二, 梁有叶. 历史极端寒冬事件研究——1892/93年中国的寒冬. 第四纪研究, 2014,34(6):1176~1185 Zhang De'er, Liang Youye. A study of the severest winter of 1892/1893 over China as an extreme climatic event in history. Quaternary Sciences, 2014,34(6):1176~1185 |
4 | 陆龙骅, 张德二. 中国年降水量的时空变化特征及其与东亚夏季风的关系. 第四纪研究, 2013,33(1):97~107 Lu Longhua, Zhang De'er. Spatio-temporal variation of annual precipitation in China and its relationship with the East Asian summer monsoon. Quaternary Sciences, 2013,33(1):97~107 |
5 | 陈星, 徐韵. 过去1000年气候模拟比较和机制分析. 第四纪研究, 2009,29(6):1115~1124 Chen Xing, Xu Yun. Climate of the past 1000 years:Simulation and mechanism. Quaternary Sciences, 2009,29(6):1115~1124 |
6 | 王江林, 杨保. 北半球及其各大洲过去1200年温度变化的若干特征. 第四纪研究, 2014,34(6):1146~1155 Wang Jianglin, Yang Bao. General characteristics of temperature changes during the past 1200 years over the North Hemisphere, the Continents and China. Quaternary Sciences, 2014,34(6):1146~1155 |
7 | 严蜜, 王志远, 刘健. 中国过去1500年典型暖期气候的模拟研究. 第四纪研究, 2014,34(6):1166~1175 Yan Mi, Wang Zhiyuan, Liu Jian. Simulation of the characteristics and mechanisms of Chinese typical warm periods over the past 1500 years. Quaternary Sciences, 2014,34(6):1166~1175 |
8 | 王志远, 刘健. 过去2000年全球典型暖期特征与机制的模拟研究. 第四纪研究, 2014,34(6):1136~1145 Wang Zhiyuan, Liu Jian. Modeling study on the characteristics and mechanisms of global typical warm periods over the past 2000 years. Quaternary Sciences, 2014,34(6):1136~1145 |
9 | 杨保, 谭明. 近千年东亚夏季风演变历史重建及与区域温湿变化关系的讨论. 第四纪研究, 2009,29(5):880~887 Yang Bao, Tan Ming. Variability of the East Asian summer monsoon and its relationship with regional temperature and moiture change during the last millennium. Quaternary Sciences, 2009,29(5):880~887 |
10 | An Z, Porter S C, Kutzbach J E et al. Asynchronous Holocene optimum of the East Asian monsoon. Quaternary Science Reviews, 2000,19(8):743~762 |
11 | Wang Y, Cheng H, Edwards R L et al. The Holocene Asian monsoon:Links to solar changes and North Atlantic climate. Science, 2005,308(5723):854~857 |
12 | 汪品先. 全球季风的地质演变. 科学通报, 2009,54(7):1113~1136 Wang Pinxian. Global monsoon in a geological perspective. Chinese Science Bulletin, 2009,54(7):1113~1136 |
13 | Fleitmann D, Burns S J, Mudelsee M et al. Holocene forcing of the Indian monsoon recorded in a stalagmite from southern Oman. Science, 2003,300(5626):1737~1739 |
14 | Neff U, Burns S J, Mangini A et al. Strong coherence between solar variability and the monsoon in Oman between 9 and 6 kyr ago. Nature, 2001,411(6835):290~293 |
15 | Wang Y J, Cheng H, Edwards R L et al. A high-resolution absolute-dated Late Pleistocene monsoon record from Hulu Cave, China. Science, 2001,294(5550):2345~2348 |
16 | Wang Y, Cheng H, Edwards R L et al. Millennial- and orbital-scale changes in the East Asian monsoon over the past 224,000 years. Nature, 2008,451(7182):1090~1093 |
17 | Cheng H, Edwards R L, Broecker W S et al. Ice age terminations. Science, 2009,326(5950):248~252 |
18 | Dykoski C, Edwards R, Cheng H et al. A high-resolution, absolute-dated Holocene and deglacial Asian monsoon record from Dongge Cave, China. Earth and Planetary Science Letters, 2005,233(1~2):71~86 |
19 | 谭明. 环流效应:中国季风区石笋氧同位素短尺度变化的气候意义——古气候记录与现代气候研究的一次对话. 第四纪研究, 2009,29(5):851~862 Tan Ming. Circulation effect:Climatic significance of the short term variability of the oxygen isotope in stalagmites from monsoonal China——Dialogue between paleoclimate records and modern climate research. Quaternary Sciences, 2009,29(5):851~862 |
20 | 姜修洋, 孔兴功, 汪永进等. 神农架三宝洞倒数第二次冰期高分辨率石笋δ13C记录. 第四纪研究, 2011,31(1):1~7 Jiang Xiuyang, Kong Xinggong, Wang Yongjin et al. A high-resolution stalagmite δ13C record from Sanbao Cave over the penultimate glaciation. Quaternary Sciences, 2011,31(1):1~7 |
21 | 董进国. 湖北三宝洞石笋生长速率及其古气候意义. 第四纪研究, 2013,33(1):146~154 Dong Jinguo. The growth and the paleoclimatic significance of stalagmites in Sanbao Cave, Hubei. Quaternary Sciences, 2013,33(1):146~154 |
22 | 吴江滢, 汪永进, 董进国. 全新世东亚夏季风演化的辽宁暖和洞石笋δ18O记录. 第四纪研究, 2011,31(6):990~998 Wu Jiangying, Wang Yongjin, Dong Jinguo. A stalagmite record of the Holocene East Asian summer monsoon from Liaoning Province. Quaternary Sciences, 2011,31(6):997~1005 |
23 | 王建明, 王建力, 李廷勇. 洞穴石笋δ18O与δ13C气候意义研究. 热带地理, 2008,28(5):395~399 Wang Jianming, Wang Jianli, Li Tingyong. The paleoclimatic significance of carbon and oxygen stable isotopes in speleothems. Tropical Geography, 2008,28(5):395~399 |
24 | 邓朝, 汪永进, 刘殿兵等."8.2ka"事件的湖北神农架高分辨率年纹层石笋记录. 第四纪研究, 2013,33(5):945~953 Deng Chao, Wang Yongjin, Liu Dianbing et al. The Asian monsoon variability around 8.2ka B.P. recorded by an annually-laminated stalagmite from Mt. Shennongjia, Central China. Quaternary Sciences, 2013,33(5):945~953 |
25 | 谭明, 侯居峙, 程海. 定量重建气候历史的石笋年层方法. 第四纪研究, 2002,22(3):209~219 Tan Ming, Hou Juzhi, Cheng Hai. Methodology of quantitatively reconstructing paleoclimate from annualy laminated stalagmites. Quaternary Sciences, 2002,22(3):209~219 |
26 | 张振球, 刘殿兵, 汪永进等. 中全新世东亚季风年至10年际气候变率:湖北青天洞5.56~4.84ka B.P. 石笋年层厚度与地球化学证据. 第四纪研究, 2014,34(6):1246~1255 Zhang Zhenqiu, Liu Dianbing, Wang Yongjin et al. Annual- to decadal-scale variability of Asian monsoon climates during Mid-Holocene:Evidence from proxies of annual bands and geochemical behaviors of a speleothem from 5.56ka B.P. to 4.84ka B.P. in Qingtian Cave, Central China. Quaternary Sciences, 2014,34(6):1246~1255 |
27 | 谭亮成, 蔡演军, 安芷生等. 石笋氧同位素和微量元素记录的陕南地区4200~2000a B.P. 高分辨率季风降雨变化. 第四纪研究, 2014,34(6):1238~1245 Tan Liangcheng, Cai Yanjun, An Zhisheng et al. High-resolution monsoon precipitation variations in southern Shaanxi, Central China during 4200~2000a B.P. as revealed by speleothem δ18O and Sr/Ca records. Quaternary Sciences, 2014,34(6):1238~1245 |
28 | Sinha A, Berkelhammer M, Stott L et al. The leading mode of Indian summer monsoon precipitation variability during the last millennium. Geophysical Research Letters, 2011,38(15):L15703 |
29 | 黄建平, 衣育红. 利用观测资料反演非线性动力模型. 中国科学(B辑), 1991,3:331~336 Huang Jianping, Yi Yuhong. Nonlinear dynamic model inversion based on observation data. Science in China (Series B), 1991,3:331~336 |
30 | 林振山, 史芳斌. 天津局地气候的反演建模及其研究. 气象学报, 1995,53(1):115~121 Lin Zhenshan, Shi Fangbin. The retrieved model of Tianjin local climate. Journal of Meteorological Research, 1995,53(1):115~121 |
31 | 罗虎明, 林振山, 李玉霞. 轨道尺度东亚夏季风的动力反演. 第四纪研究, 2013,33(3):603~609 Luo Huming, Lin Zhenshan, Li Yuxia. The dynamic inversion of orbital-scale East Asian summer monsoon. Quaternary Sciences, 2013,33(3):603~609 |
32 | Liu H Y, Lin Z S, Qi X Z et al. Possible link between Holocene East Asian monsoon and solar activity obtained from the EMD method. Nonlinear Processes in Geophysics, 2012,19(4):421~430 |
33 | Beer J, Mende W, Stellmacher R. The role of the sun in climate forcing. Quaternary Science Reviews, 2000,19(1):403~415 |
34 | Agnihotri R, Dutta K, Soon W. Temporal derivative of Total Solar Irradiance and anomalous Indian summer monsoon:An empirical evidence for a sun-climate connection.Journal of Atmospheric and Solar-Terrestrial Physics, 2011,73(13):1980~1987 |
35 | Kodera K. Solar influence on the Indian Ocean monsoon through dynamical processes. Geophysical Research Letters, 2004,31(24):L24209 |
36 | Prell W L, Kutzbach J E. Sensitivity of the Indian monsoon to forcing parameters and implications for its evolution. Nature 1992,360(6405):647~652 |
37 | Huybers P. Early Pleistocene glacial cycles and the integrated summer insolation forcing. Science, 2006,313(5786):508~11 |
38 | 刘健, 陈星, 郑景云等. 千年气候模拟与中国东部温度重建序列的比较研究. 科学通报, 2005,50(20):2251~2255 Liu Jian, Chen Xing, Zheng Jingyun et al. Comparative study on millennium climate simulation and eastern Chinese temperature reconstruction. Chinese Science Bulletin, 2005,50(20):2251~2255 |
39 | Mann M E, Cane M A, Zebiak S E et al. Volcanic and solar forcing of the tropical pacific over the past 1000 years. Journal of Climate, 2005,18(3):447~456 |
40 | Lean J, Beer J, Bradley R. Reconstruction of solar irradiance since 1610:Implications for climate change. Geophysical Research Letters, 1995,22(23):3195~3198 |
41 | Lean J, Rind D. Earth's response to a variable Sun. Science, 2001,292(5515):234~236 |
42 | Shindell D T, Schmidt G A, Mann M E et al. Solar forcing of regional climate change during the Maunder Minimum. Science, 2001,294(5549):2149~2152 |
43 | Magny M. Holocene climate variability as reflected by mid-European lake-level fluctuations and its probable impact on prehistoric human settlements. Quaternary International, 2004,113(1):65~79 |
44 | Hong Bing, Lin Qinghua, Hong Yetang. Interconnections between the Asian monsoon, ENSO, and high northern latitude climate during the Holocene. Chinese Science Bulletin, 2006,51(18):2169~2177 |
45 | Gupta A K, Anderson D M, Overpeck J T. Abrupt changes in the Asian southwest monsoon during the Holocene and their links to the North Atlantic Ocean. Nature, 2003,421(6921):354~357 |
46 | Hong Y T, Hong B, Lin Q H et al. Synchronous climate anomalies in the western North Pacific and North Atlantic regions during the last 14,000 years. Quaternary Science Reviews, 2009,28(9~10):840~849 |
47 | Hong Y T, Hong B, Lin Q H et al. Correlation between Indian Ocean summer monsoon and North Atlantic climate during the Holocene. Earth and Planetary Science Letters, 2003,211(3~4):371~380 |
48 | Hong Y T, Hong B, Lin Q H et al. Inverse phase oscillations between the East Asian and Indian Ocean summer monsoons during the last 12000 years and paleo-El Niño. Earth and Planetary Science Letters, 2005,231(3~4):337~346 |
49 | Srivastava A K, Rajeevan M, Kulkami R. Teleconnection of OLR and SST anomalies over Atlantic Ocean with Indian summer monsoon. Geophysical Research Letters, 2002,29(8):125~1-125~4 |
50 | Parker A G, Goudie A S, Stokes S et al. A record of Holocene climate change from lake geochemical analyses in southeastern Arabia. Quaternary Research, 2006,66(3):465~476 |
51 | Wang Shaowu, Ge Quansheng, Wang Fang et al. Abrupt climate changes of Holocene. Chinese Geographical Science, 2013,23(1):1~12 |
52 | Asmerom Y, Polyak V, Burns S et al. Solar forcing of Holocene climate:New insights from a speleothem record, Southwestern United States. Geology, 2007,35(1):1~4 |
53 | 李晓东, 王绍武, 黄建平. 火山活动对气候影响的数值模拟研究. 应用气象学报, 1994,5(1):90~97 Li Xiaodong, Wang Shaowu, Huang Jianping. Numerical simulation of volcanic impact on climate. Quarterly Journal of Applied Meteorology, 1994,5(1):90~97 |
54 | 杨冬红, 杨学祥. 全球气候变化的成因初探. 地球物理学进展, 2013,28(4):1666~1677 Yang Donghong, Yang Xuexiang. Study on cause of formation in Earth's climatic changes. Progress in Geophysics, 2013,28(4):1666~1677 |
55 | 郭正府, 刘嘉麒. 火山活动与气候变化研究进展. 地球科学进展, 2002,17(4):595~604 Guo Zhengfu, Liu Jiaqi. Research advance in effect of volcanism on climate changes. Advance in Earth Sciences, 2002,17(4):595~604 |
56 | Doe B R. The past is the key to the future. Geochimica et Cosmochimica Acta, 1983,47(8):1341~1354 |
57 | 吴国雄, 张永生. 青藏高原的热力和机械强迫作用以及亚洲季风的爆发Ⅰ. 爆发地点. 大气科学, 1998,22(6):826~838 Wu Guoxiong, Zhang Yongsheng. Thermal and mechanical forcing of the Tibetan Plateau and the Asian monsoon onset. Part Ⅰ:Situating of the onset. Chinese Journal of Atmospheric Sciences, 1998,22(6):826~838 |
58 | Gray V. Climate Change 2007:The Physical Science Basis. Summary for Policy Makers. International Panel on Climate Change. Fourth Assessment Report, IPCC Secretariat, 2007,54(4):44~45 |
59 | Sinha A, Cannariato K G, Stott L D et al. A 900-year(600 to 1500A.D.) record of the Indian summer monsoon precipitation from the core monsoon zone of India. Geophysical Research Letters, 2007,34(16):L16707 |
60 | Steinhilbera F, Abreu J A, Beer J et al.9,400 years of cosmic radiation and solar activity from ice cores and tree rings.Proceedings of the National Academy of Sciences of the United States of America, 2012,109(16):5967~5971 |
61 | Yan H, Sun L, Wang Y et al. A record of the Southern Oscillation Index for the past 2,000 years from precipitation proxies. Nature Geoscience, 2011,4(9):611~614 |
62 | MacDonald G M. Variations in the Pacific Decadal Oscillation over the past millennium. Geophysical Research Letters, 2005,32(8):L08703 |
63 | Etheridge D, Steele L, Francey R et al. Atmospheric methane between 1000 AD and present:Evidence of anthropogenic emissions and climatic variability. Journal of Geophysical Research:Atmospheres, 1998,103(D13):15979~15993 |
64 | Thornalley D J, Elderfield H, McCave I N. Holocene oscillations in temperature and salinity of the surface subpolar North Atlantic. Nature, 2009,457(7230):711~714 |
65 | Staubwasser M,Sirocko F, Grootes P M et al. South Asian monsoon climate change and radiocarbon in the Arabian Sea during Early and Middle Holocene. Paleoceanography, 2002,17(4):15~1-15~12 |
66 | Thompson L G, Yao T, Davis M E et al. Holocene climate variability archived in the Puruogangri ice cap on the central Tibetan Plateau. Annals of Glaciology, 2006,43(1):61~69 |
67 | Kobashi T, Kawamura K, Severinghaus J P et al. High variability of Greenland surface temperature over the past 4000 years estimated from trapped air in an ice core. Geophysical Research Letters, 2011,38(21):L21501 |
68 | Mulvaney R, Abram N J, Hindmarsh R C et al. Recent Antarctic Peninsula warming relative to Holocene climate and ice-shelf history. Nature, 2012,489(7414):141~144 |
69 | Zielinski G A, Mershon G R. Paleoenvironmental implications of the insoluble microparticle record in the GISP 2(Greenland) ice core during the rapidly changing climate of the Pleistocene-Holocene transition. Geological Society of America Bulletin, 1997,109(5):547~559 |
70 | 李荣华, 冯果忱. 微分方程的数值解法. 北京:高等教育出版社, 1996. 54~62 Li Ronghua, Feng Guochen. Numerical Analysis of Differential Equations. Beijing:Higher Education Press, 1996. 54~62 |
71 | Griggs D J, Noguer M. Climate change 2001:The scientific basis. Contribution of Working Group I to the Third Assessment Report of the Intergovernmental Panel on Climate Change. Weather, 2001,57(8):267~269 |
72 | Sarthi P P, Dash S K, Mamgain A. Possible changes in the characteristics of Indian summer monsoon under warmer climate. Global and Planetary Change, 2012,92~93(5):17~29 |
73 | Zhang Y, Wallace J M, Battisti D S. ENSO-like interdecadal variability:1900~93. Journal of Climate, 1997,10(5):1004~1020 |
74 | 赵侃. 贵州董哥洞近1000年石笋年纹层年代学与同位素气候重建. 南京:南京师范大学博士学位论文, 2011. 1~146 Zhao Kan. Dongge Cave Stalagmites Laminated Chronology and Climate Reconstruction in the Last Millennium. Nanjing:The Doctoral Dissertation of Nanjing Normal University, 2011. 1~146 |
75 | Zhang R, Delworth T L. Simulated tropical response to a substantial weakening of the Atlantic thermohaline circulation. Journal of Climate, 2005,18(12):1853~1860 |
Abstract
Researches on paleoclimate driving mechanism during the last millennium allow us to place the warming of the 20th century within a historical context. The mechanism also plays a significant role in understanding the evolution law of the climate system and predicting the future climate variability. Indian Summer Monsoon(ISM) is the most prominent part of the world's monsoon systems, which primarily affects India and its surrounding water bodies. Solar radiation and volcanic impact are acknowledged external forcing of ISM. Many researches have shown that there are close relevance between ISM, El Niño Southern Oscillation(ENSO), Pacific Decadal Oscillation(PDO), North Atlantic Sea Surface Temperature(SST) and so on. Someone proposed that the mechanical and thermodynamic force of Qinghai-Tibet Plateau may be incentives of the Asian summer monsoon outburst. In addition, volcanic eruption may also be an nonnegligible factor of ISM. However, our understanding of the ISM variability on centennial time scales remains insufficient. Cave stalagmites δ18O is one of the most important high-resolution proxies of paleo-monsoon. Jhumar Cave is located near the town of Jagdalpur in central India(18°52'N, 81°52'E; 600m a.s.l.), where annual precipitation variability is dominated(70%~80%) by monsoon precipitation from July to September. As the annual precipitation of Jhumar Cave is highly related to regional monsoon precipitation, stalagmites from this cave can be used to indicate the ISM variability. In this study, a nonlinear statistical-dynamical inversion model was used to quantitatively analyze the driving mechanism of ISM based on the stalagmite data of Jhumar Cave. Traditionally, the statistical models work best in data processing, especially in the aspects of regression, fitting and correlation analysis. However, they cannot reveal the internal dynamic mechanism of data as the mechanism models do. Our nonlinear statistical-dynamical inversion model, which combines both the advantages of the traditional statistical models and dynamical models, can quantitatively analyze the driving mechanism of the paleoclimate system by making full use of the observation data. Based on this model, the following results are obtained:(1) The ISM system is a complex nonlinear dynamical system.(2) Before the Industrial Revolution, ISM is mainly driven by the North Atlantic SST, then the interaction among the greenhouse gas(N2O and CO2) concentration, the Arabian Sea surface temperature, ENSO and the total solar irradiance and so on. After the Industrial Revolution, the interaction among the greenhouse gas(CH4, N2O and CO2) concentration, the North Atlantic Sea surface temperature, the total solar irradiance, ENSO and North polar temperature and so on become the main driving factors of ISM. (3) The linear term of CH4 and N2O are two driving factors of ISM after 1800A.D., but the nonlinear interaction between the two factors is stabilizing factor. In general, the North Atlantic play an important role in affecting the ISM variability before 1800A.D. and the greenhouse gases play an important role after 1800A.D.